When Dead Stars Collide!
In October 2017, for the first time, astronomers observed light and gravitational waves from the same source.
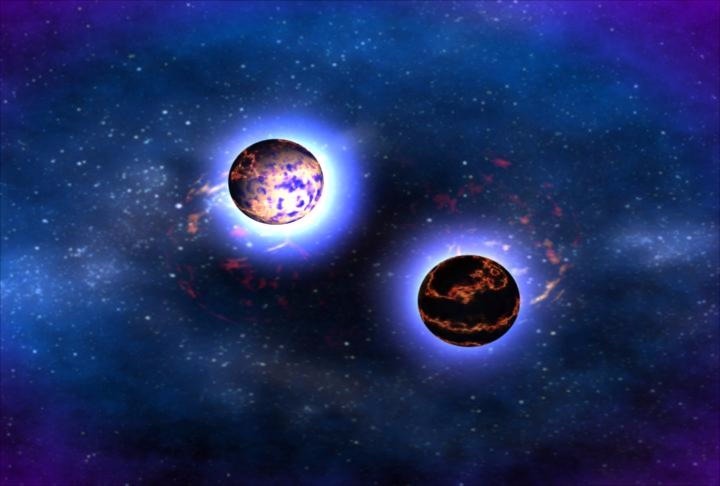
4 min read
When Dead Stars Collide!
Gravity has been making waves — literally. In October 2017, the Nobel Prize in Physics was awarded for the first direct detection of gravitational waves two years earlier. Also in that month, astronomers announced a huge advance in the field of gravitational waves: For the first time, they had observed light and gravitational waves from the same source. Let’s look at what happened.
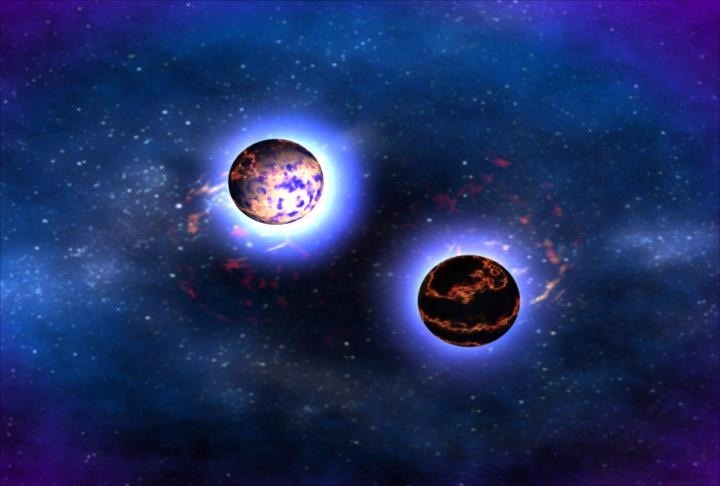
There was a pair of orbiting neutron stars in a galaxy (called NGC 4993). Neutron stars are the crushed leftover cores of massive stars (stars more than 8 times the mass of our sun) that long ago exploded as supernovae. There are many such pairs of binaries in this galaxy, and in all the galaxies we can see, but something special was about to happen to this particular pair.
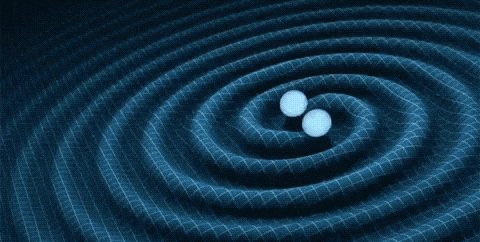
Each time these neutron stars orbited, they would lose a teeny bit of gravitational energy to gravitational waves. Gravitational waves are disturbances in space-time — the very fabric of the universe — that travel at the speed of light. The waves are emitted by any mass that is changing speed or direction, like this pair of orbiting neutron stars. However, the gravitational waves are very faint unless the neutron stars are very close and orbiting around each other very fast.
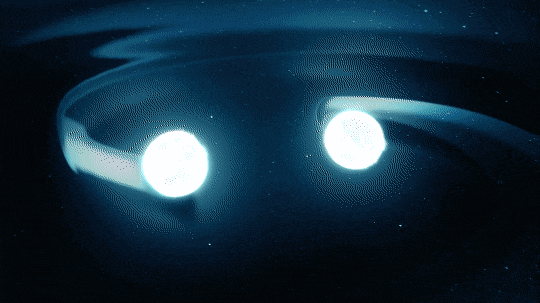
The teeny energy loss caused the two neutron stars to get a teeny bit closer to each other and orbit a teeny bit faster. After hundreds of millions of years, all those teeny bits added up, and the neutron stars were very close. So close that … BOOM! … they collided. And we witnessed it on Earth on August 17, 2017.
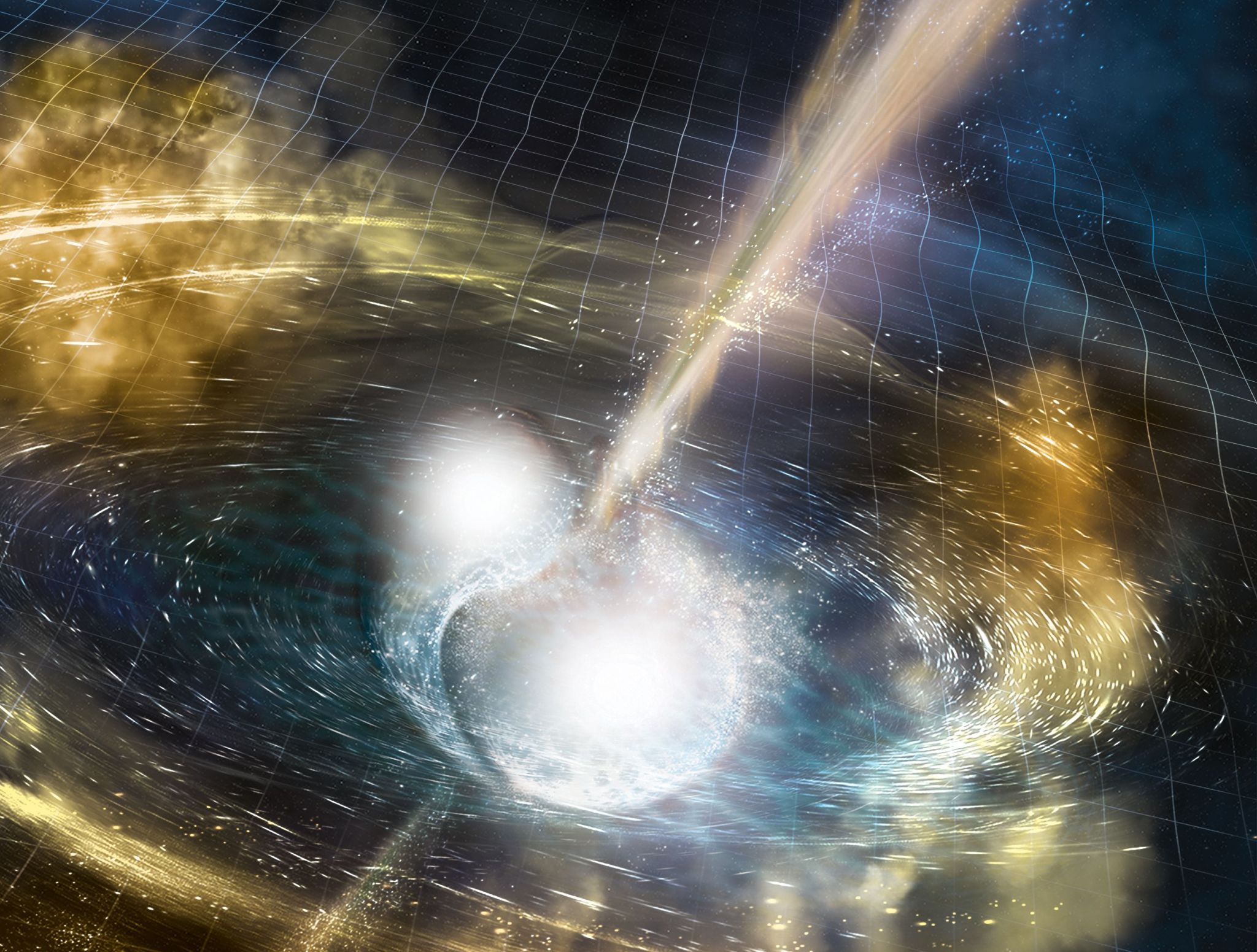
A couple of very cool things happened in that collision, and we expect they happen in all such neutron-star collisions. Just before the neutron stars collided, the gravitational waves were strong enough and at just the right frequency that the National Science Foundation’s Laser Interferometer Gravitational-Wave Observatory (LIGO) and European Gravitational Observatory’s Virgo could detect them. Just after the collision, those waves quickly faded out because there are no longer two things orbiting around each other!
LIGO and Virgo are ground-based detectors waiting for gravitational waves to pass through their facilities on Earth. When it is active, it can detect them from almost anywhere in space.
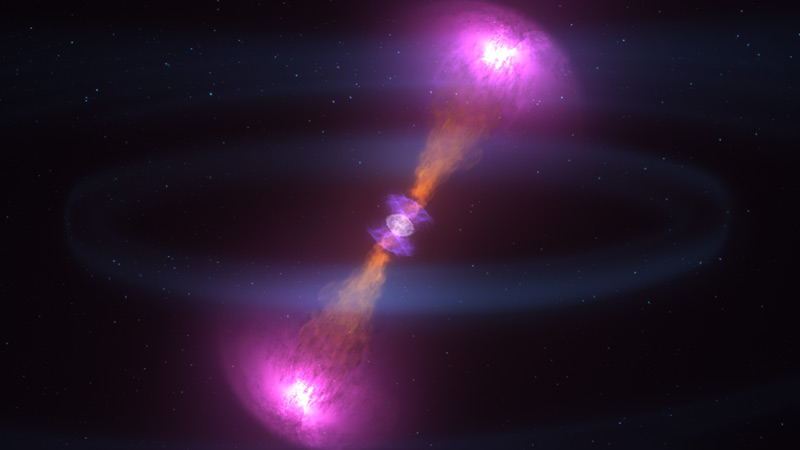
The other thing that happened was what we call a gamma-ray burst. When they get very close, the neutron stars break apart and create a spectacular, but short, explosion. For a couple of seconds, our Fermi satellite saw gamma rays from that explosion. Fermi’s Gamma-ray Burst Monitor is one of our eyes on the sky, looking out for such bursts of gamma rays that scientists want to catch as soon as they’re happening.
And those gamma rays came just 1.7 seconds after the gravitational wave signal. The galaxy this occurred in is 130 million light-years away, so the light and gravitational waves were traveling for 130 million years before we detected them.
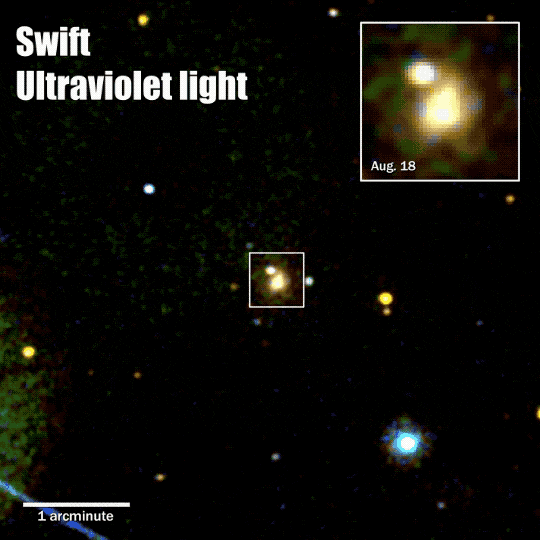
After that initial burst of gamma rays, the debris from the explosion continued to glow, fading as it expanded outward. Our Swift, Hubble, Chandra, and Spitzer telescopes, along with a number of ground-based observatories, were poised to look at this afterglow from the explosion in ultraviolet, optical, X-ray, and infrared light. Such coordination between satellites is something that we’ve been doing with our international partners for decades, so we catch events like this one as quickly as possible and in as many wavelengths as possible.
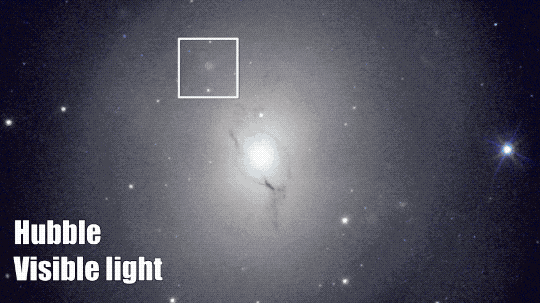
Astronomers have thought that neutron star mergers were the cause of one type of gamma-ray burst — a short gamma-ray burst, like the one they observed on August 17. It wasn’t until we could combine the data from our satellites with the information from LIGO/Virgo that we could confirm this directly.
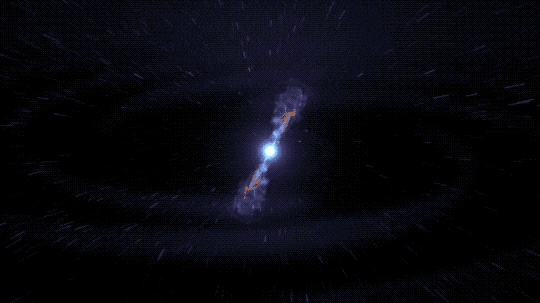
That event began a new chapter in astronomy. For centuries, light was the only way we could learn about our universe. Now, we’ve opened up a whole new window into the study of neutron stars and black holes. This means we can see things we could not detect before.
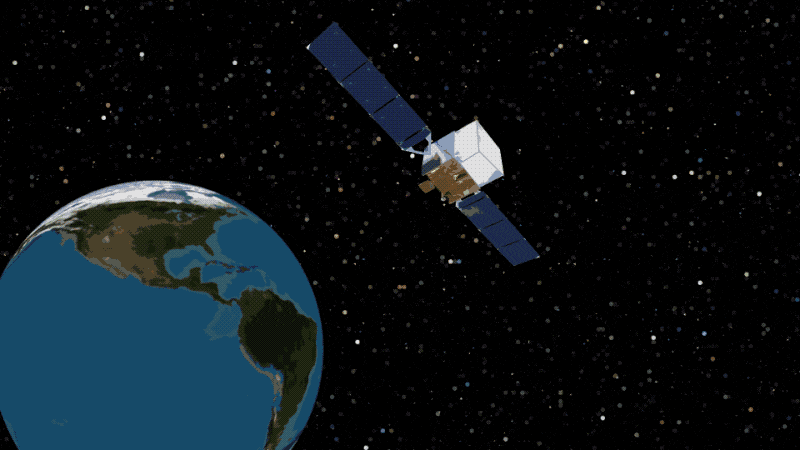
The first LIGO detection was of a pair of merging black holes. Mergers like that may be happening as often as once a month across the universe, but they do not produce much light because there’s little to nothing left around the black hole to emit light. In that case, gravitational waves were the only way to detect the merger.
The neutron star merger, though, has plenty of material to emit light. By combining different kinds of light with gravitational waves, we are learning how matter behaves in the most extreme environments. We are learning more about how the gravitational wave information fits with what we already know from light — and in the process we’re solving some long-standing mysteries!
Share
Details
Related Terms
What's Your Reaction?
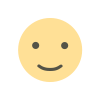
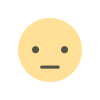
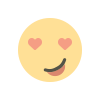
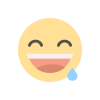
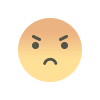
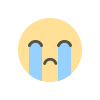
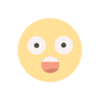